Innovative Measurement and Photon Science
Quantifying structure and dynamics from the atomic to the mesoscopic scale
Any understanding of chemical, physical and biological structure and dynamics is fundamentally linked to our ability to characterise any material or process with the highest possible spatio-temporal resolution. All molecular science, if analytical or synthetic, depends heavily on the characterisation of sample identity in terms or both chemical composition and structure, sample stability and in case of mechanistic studies, temporal profiles of physical or chemical parameters. As our measurement accuracy of these sample properties and external variables improves, so does our ability to fine-tune reactions to achieve desired outcomes as well as our understanding of the molecular origin of many chemical and physical phenomena and our abilities to affect or clone them for our purposes. Widening the application of existing measurement protocols and techniques to new problems as well as developing entirely novel experimental, computational and analytical approaches to provide hitherto inaccessible information or data of previously unknown sensitivity or resolution will hence benefit all branches of the molecular sciences.
We use and develop techniques as diverse as spectroelectrochemistry, high-resolution microscopies and laser tweezers, Surface Force Balance measurements, gas and condensed phase optical cavity methodologies, Nuclear and Electron Paramagnetic Magnetic Resonance, X-ray and neutron diffraction and Mass Spectrometry.
Fundamental branches of measurement development and application span the following areas:
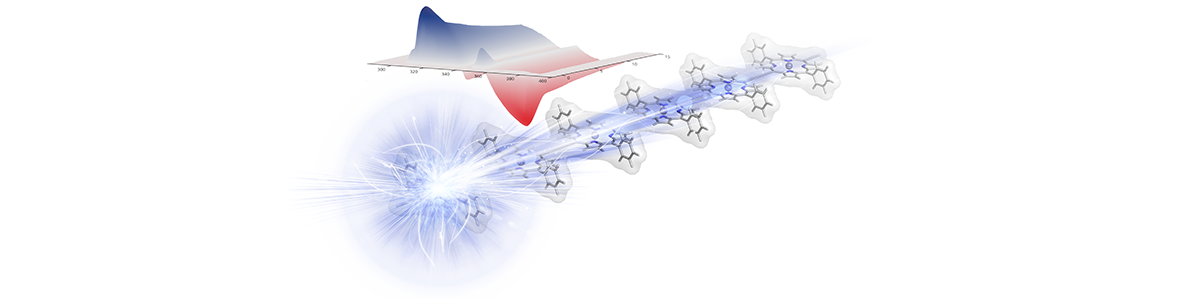
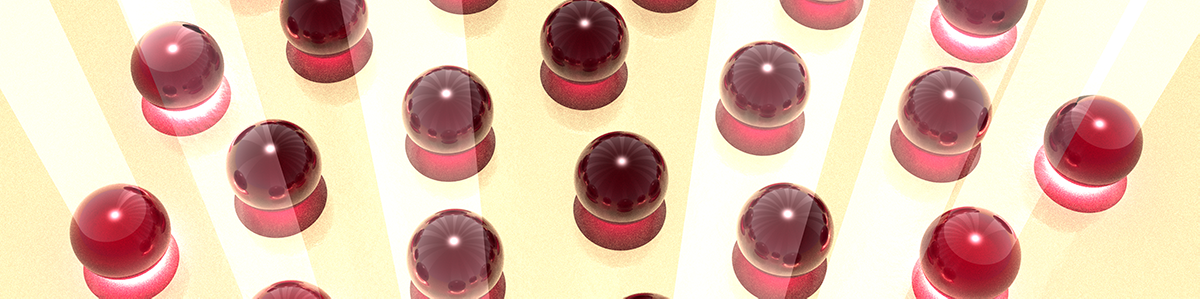
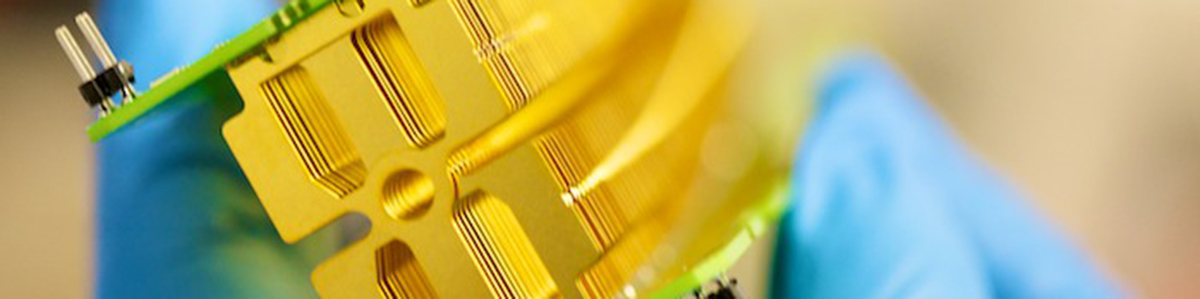
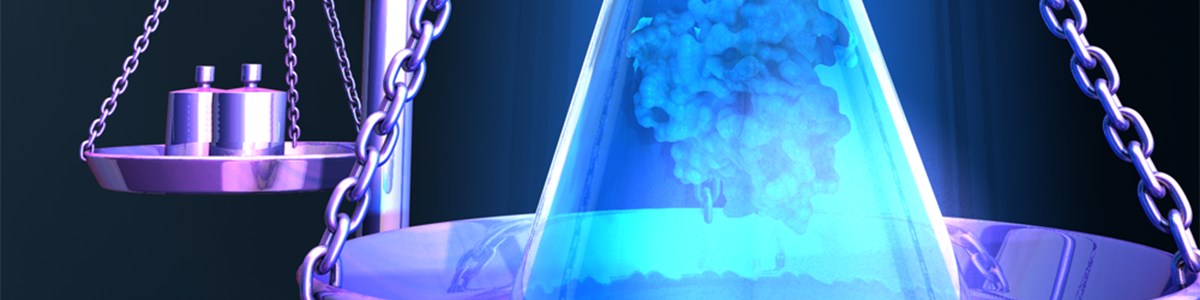
Our expertise lies in the measurement of forces in simple and complex fluids, as well as in ionic liquids, and in the measurement of interaction energies between entities at the molecular scale. To this end we apply a variety of techniques such the Surface Force Balance, (Holographic) Optical Tweezers and the newly developed Electrostatic Fluidic Trap. These approaches permit us to measure ultra-small forces at very small scales, locally in 3D and time-resolved. Such quantitative measurements shine light on the properties of liquids in super-confinement, relevant for example in lubrication, on the strengths of novel materials, and on forces at play in biology. The ability to perform high precision measurements using molecules as probes of interaction energies is paving the way towards the discovery of previously unanticipated forces in interparticle and intermolecular interactions in the fluid phase.
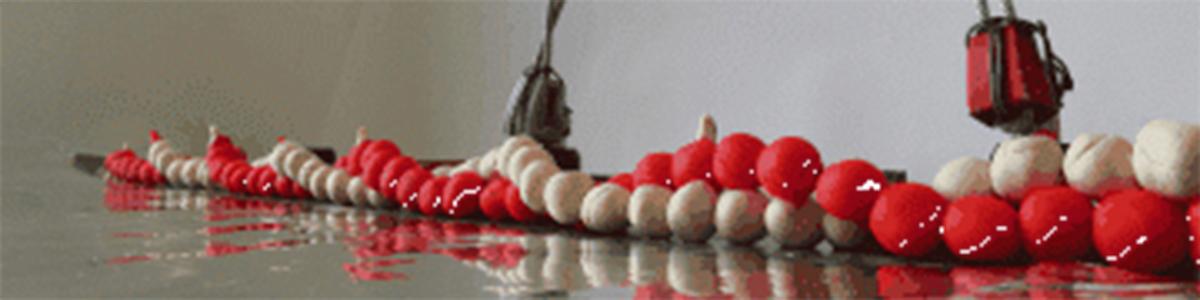
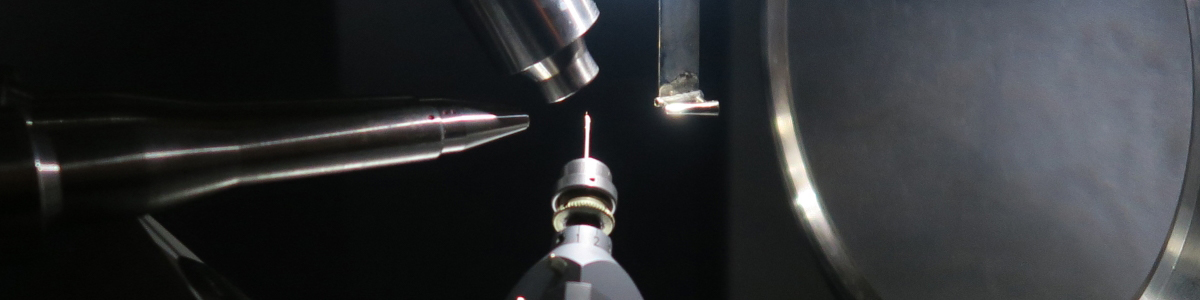
Oxford Electrochemistry ranges broadly and deeply across fundamental and applied electrochemistry and spectro-electrochemistry to biology, fuel cells, chemical sensors and electrosynthesis. In all these areas the design, validation, modelling and application of new experiments and instruments is at the heart of our research whether it is on enzymes, nanoparticles, molecules or whatever. The work is generally bottom-up and characterised by a rigorous physic-chemical basis.
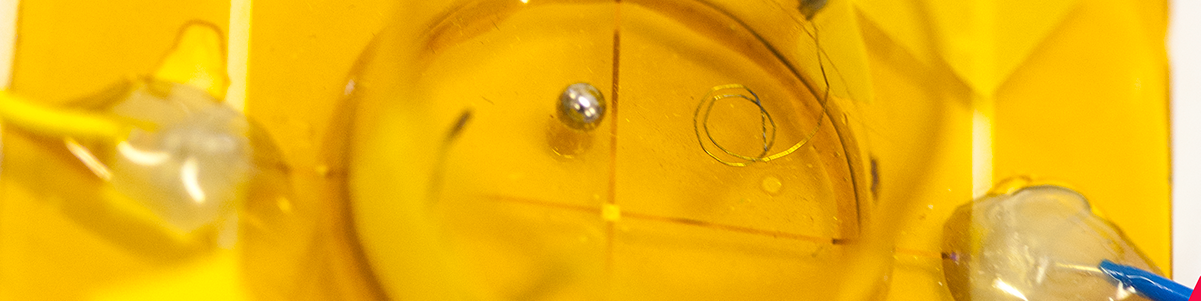